Creatures from the Black Lagoon: Lessons in the Diversity and Evolution of Eukaryotes
by Scott Dawson
Ive organized this lecture into
several parts, beginning with a history of biological classification
of life especially in the context of microorganisms. I also want to talk about the diversity and lifestyle of microbes
(protists in particular) that live without oxygen and why this is
relevant to the evolution of life especially with respect to
eukaryotic evolution. Then Im going to discuss how the majority
of microbial life is yet to be discovered, and how one might find new
microbes of interest to the study of evolution. And lastly, Ill
talk about some new local monsters we've found microbial
creatures from the Black Lagoon, where they live (just in case you
want to check them out), and why they could change our understanding
of eukaryotic evolution.
So, to start, I think when I first I learned
about evolution there was always an implied "march of progress," or a
linear progression from one form of life to a next, higher and
higher, up the evolutionary ladder. This notion usually begins with
some primordial soup progressing to bacteria to
protist to plant to animal bugs to us. But
we know this is false. Evolution is branching or at least
thats been the thinking in the past 150 years. Ill be
talking more about this, but in order to do good research in
evolution, we need to examine where these evolutionary "stories" come
from what are the assumptions? Im going to try to
address several of these assumptions and misconceptions by
showcasing some of the scientists who formed the basis of the
classification of microorganisms.
Aristotle
Let's start in ancient Greece with Aristotle in addition to many other
contributions to science, he was the first to articulate a dichotomy
of life, classifying all life as being either plants or animals. This
ancient world view of biology persists today, and misinforms our
perspective in terms of evolution, not to mention the structuring of
scientific research (like botany departments and zoology
departments). It was a few thousand years later that the microscope was
invented, and animal/vegetable/or mineral types of classification
were hardly enough to classifiy the new microorganisms, first
called "animacules." Microbes are often non-descript rods, or
spheres. How then does one classify microbes, particularly when they
don't really look different from one another like plants look
different from animals? More about how to do that later, but on to
more history.
|
|
Carolus Linnaeus
Linnaeus lived in the 18th Century and created the familiar hierarchical
classification scheme of life: kingdom, family, class, order,
family, genus and species. Again, this classification scheme is based on
how organisms looked essentially their phenotype. Although this
heirarchy within the plant and animal kingdoms was an improvement
an attempt to standardize classification whose legacy persists today
there were still no good working definitions of "kingdoms" or
"species" which could apply to microorganisms when they were
discovered. Even today the conception of a species of bacteria is
hard to define, in the same way an animal species is
defined.
|
|
|
Charles Darwin
We are all probably familiar with Charles Darwin, 19th century
father of natural selection and evolution, but he also called for a
framework or geneology of organisms in order to study evolution.
Darwin helped to develop the concept of a geneology of life with
branching lineages. But we attribute the conception of evolutionary
relationships among organisms with a tree a geneology to Ernst
Haeckel at roughly the same time.
|
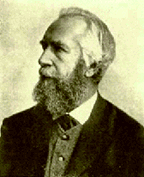
|
|
Ernst Haeckel
Ernst Haeckel also worked in the mid-19th century. Haeckel was
the first to coin the term "protist" or "Protista," although his
definition also included the bacteria.
|
Haeckel's Tree of Life
Ernst Haeckel is attributed to be the first
to describe the evolutionary relationships among living organisms, a geneology of life, as analogous to a tree. Haeckel, interestingly,
described all living things as falling into not just two kingdoms
(plants and animals), but also a third kingdom for microorgansims the Protista. There are several other interesting features of this
vision for example, Haeckel postulated a common origin for all
life (plants, animals and microbes). This is still a common
assumption, but it makes sense with modern molecular evidence as well.
OK, let's fast forward a hundred years or so. How do we classify
microbes today, especially when they have no particular morphology or shape to speak of. Scientists had all but given up on
classifying microbes based on their evolutionary relationships (a
phylogeny) until Carl Woese.
|
|
|
The Woesian Revolution
Woese based his classification on molecules,
not how organisms look or act. This transition from classification
based on phenotype (taxonomy) to one based on genotype enabled him to
determine the evolutionary relationships (a phylogeny) among bacteria something other researchers had all but given up on. Woese's work was founded on the principle suggested in 1965 that "molecules are
documents of evolutionary history." Basically, DNA can be thought of
as molecular fossils. At the University of Illinios in the late 1970s, Woese wanted to
determine evolutionary relationships among microorganisms, and in
the process, he and colleagues discovered a huge split in the
"prokaryotes" as big a genetic difference as that between prokaryotes
and eukaryotes. Woese orignally thought that these were primitive
organisms and so called them the Archaea. Later studies showed that
the Archaea were actually more related to the eukaryotes in many
aspects that to the bacteria. So, rather than five kingdoms of life,
Woese argued for three domains (Eucarya, Archaea, and Bacteria). This
conception of three domains of life and two domains of prokaryotes, rather
than the standard prokaryote/eukaryote dichotomy, really shook up the
study of the evolution of life. So how did Woese arise at this
remarkable conclusion? First he needed a molecule to study from all
organisms ribosomal RNA (rRNA).
|
Why use rRNA?
Ribosomal RNA (rRNA) is an RNA component of
the ribosome, the cellular machine which translates the DNA genetic
code to amino acids, and subsequently, proteins. The rRNA genes are
ubiquitous in all life, being conserved enough to identify, yet
containing enough variabilty to determine evolutionary relationships.
But Woese has had a hard time selling this idea, mostly because
everyone has been trained to view life as prokaryotes/eukaryotes,
plants/animals, or the updated version of that &emdash; the five kingdom
view.
|
|
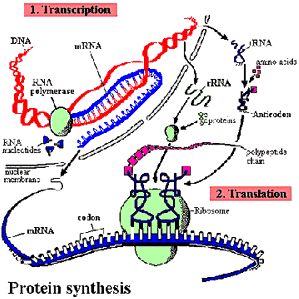
|
Five Kingdoms or Three Domains?
In the 1960s, Whittaker added one kingdom (fungi) to Aristotles's
view of life (plants and animals) but still relegated all microbes to
either the Protista (eukaryotic microbes) or the Monera (bacteria).
Something from this figure (and view) should be obvious. Why should
we emphasize macroscopic life over microscopic life? In terms of life
on Earth, we still live in an age of microbes. For the majority of
geologic time, some 3-4 billion years, life has evolved and at least three
billion has been solely microbial.
|
|
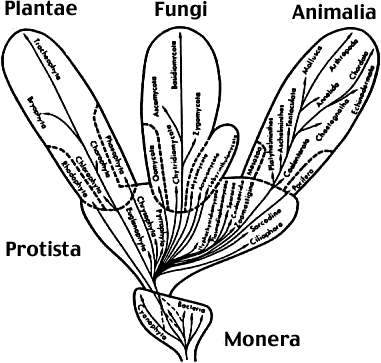
|
The five-kingdom view is still taught, as
I'm sure many of you know. Carl Woese started a revolution in the
classification of life, much like Copernicus did with his idea that the
Sun doesn't revolve around the Earth. We, as humans, possess a
lot of unique characteristics such as the ability to understand
the world we live in. But we are not the pinnacle of evolution nothing is. Evolution is about change through time, not about
ranking life based on some criterion. Besides, since the bulk of
genetic diversity of life on Earth is been microbial (note where we
are in Woese's tree), shouldn't they get attention simply for that
reason? If you take nothing else from my talk, stop thinking about
biology in terms of five kingdoms.
|
|
|
Since Woese's early trees, we've added a lot of sequences as you can see
in the figure to the left. I'd like to switch now from talking about
microbes in general, to specfically eukaryotic microbes (that is,
microbial cells which possess a membrane-bound nucleus and tend to be
unicellular). What's the current picture of eukaryotic
phylogeny?
|
The Evolution of the Eukaryotes
As in the prokaryotic world, one can see
from this schematic tree of the eukaryotes (below), that the majority of
eukaryotic life is, in fact, microbial (protists). There is far more
phenotypic and genotypic diversity in all the protist groups combined
than within the plant, animal or fungal kingdoms. How many kingdoms
of eukaryotes are there? This is still largely unknown. If we use the genetic measure, we come up with roughly 70 eukaryotic kingdoms, only some of which are shown here. Further, because of our
bias toward macroscopic life, most of the biology of these protists
is largely unknown. How did the eukaryotes evolve? To understand
that, we need to visualize the early Earth.
II. Life in anoxic worlds
Early Earth
We know from the geologic record that the early Earth was a fireball and
the atmosphere was quite different from what it is today in fact,
no oxygen was present. All oxygen was produced biogenically by microorganisms several billion years after the earth was formed. So for roughly half of Earth's history, there was no oxygen atmosphere. When microbes began producing oxygen in such quantities that it began to saturate the atmosphere, it was the biggest
environmental catastrophe in Earth's history.
Some of the oldest fossils belong to what look like photosynthetic bacteria bacteria which breathe carbon dioxide and exhale oxygen. So this process happened early in Earth's history.
Microbes made the world. Think about it oxygen is actually quite poisonous, generating hydrogen peroxides and oxygen radicals. Many microbes don't have a way of dealing with poisonous oxygen. So they avoided it . . . and they still do today.
Fossil Redbeds
This is a rock from a fossil redbed. The
red color derives from oxidized iron, like rust. When the percentage
of oxygen in the atmosphere became high enough, iron mineral deposits
became oxidized (red). Reduced iron compounds like iron sulfides in
contemporary anoxic environments look black. How did this happen?
Microbes. Specifically, bacteria called cyanobacteria (or blue-green
algae) they use light as energy, breathe carbon dioxide and generate
oxygen.
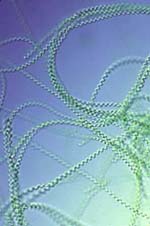
Cyanobacteria
This is one example of a cyanobacterium, Spirulina. Spirulina is pretty
common in many aquatic habitats. If you've ever seen a green scum on
the surface of soils/sediments, that is cyanobacteria. Think of how
many cells you would need to completely change the composition of the
Earth's atmosphere, as happened a few billion years ago.
Cyanobacteria are found all over the world. In fact, in Berkeley you
can find them in grocery stores as . . . Spirulina Smoothies!
Evolution of Oxygen
This graph shows the percentage of oxygen in the atmosphere over time and
indicates when red beds would have formed. The first appearance of
red beds is at two billion years, which is also the oft-cited age of
eukaryotes (cells with a membrane-bound nucleus). Why? The oldest
eukaryotic fossils are coincidentally about the same age. But do
eukaryotes require oxygen? We were all taught about simple cells like bacteria which use glycolysis or fermentation
alone to generate ATP (the energy currency of the cell). Then as
evolution progressed, eukaryotes developed the ability to harness
oxygen via the mitochondrion, the energy powerhouse of the cell. This
is another misconception. First, bacteria invented oxidative
respiration eukaryotes just acquired the ability, by acquiring a
bacteria cell by endosymbiosis this later became the mitochondrion.
Also, many eukaryotes do live prosperous lives without oxygen (or
without mitochondria for that matter). Here's one below (Giardia), that
hopefully no one has had a personal relationship with:
Giardia is an aquatic protist, and a parasite that major cause of dysentery
world wide. Hopefully no one here has encountered it while camping.
Giardia has no mitochondrion and it likes to avoid oxygen. Why?
Oxygen is an abundant source of energy, but in many aquatic
environments, it gets quickly used up by microorganisms. Many
bacteria, however, can respire or "breathe" alternatives to oxygen
sulfur, hydrogen, nitrogen for example. These bacteria are
called anaerobes (more on this later). Environments lacking oxygen
(anoxic) harbor many of these types of bacteria &emdash; billions per
teaspoon of sediment or muck. In many cases, the anoxic environments
harbor more microorgansisms than oxic ones. Giardia survives by
eating anaerobic bacteria in these rich anoxic environments. But
where on Earth are these "extreme" anoxic environments? Where would
one go to find them?
Contemporary anoxic worlds
Virtually anywhere! Check it out the
Black sea is the largest land-locked anoxic basin. Can we find more
organisms like Giardia which might still live in anoxic worlds, and
still be unknown to us? How could we do it?
III. Uncultivated Microbial Eukaryotes
What is the extent of eukaryotic diversity?
How do we find life on Earth? Why should we
care? Well, in terms of studying evolution, our knowledge of modern
eukaryotes informs our knowledge of their evolution. An incomplete
understanding of diversity of today's eukaryotes results in
incomplete evolutionary trees.
Characteristics of eukaryotes that live without oxygen
Most known anaerobic protists are pathogens. One could argue that the only
reason we know about the ones we do is becuase they affect us they
cause disease. Anaerobic protists have evolved multiple times (which
is termed "polyphyletic") but since the most basal eukaryotes
are anaerobes, we believe the original eukaryotic cell evolved in an
anoxic world. How might we look for eukaryotes still alive today,
which might be more genetically closer to those ancestral types, than
to more recently evolved groups like us?
Identifying protists by microscopy
One way we could do this would be by microscopy. Antoine van
Leeuwenhoek, a lay person (not a card-carrying scientist), really began the study of microbiology in the 17th century with the use of one of the first microscopes. van Leewenhoek described the first microorganisms he called them "animalcules."
People still identify microbes based solely on microscopic descriptions today. These descriptions can read like field guides. Though our microscopes have gotten a lot more sophisticated since van Leewenhoek (e.g., electron microscopes), are
there other ways to find these relict eukaryotes?
Identifying protists by cultivation
Well, we could grow them. We know Louis Pasteur for a lot of work with
microbiology, such as germ theory and pasteurization. But he
was also the first to describe organisms which were able to grow in
sealed flasks without "air." This was truly a first, as people at
that time believed that all organisms needed air, meaning oxygen, to
grow.
But we encounter problems when we rely only on
visualizing or growing organisms in order to identify them. First and
foremost, it can be really hard! Think if we had to grow every animal
in the zoo or every plant in the forest in order to know they were
there. Plus, we don't do a really good job at growing most microbes.
It's estimated that only about 1% of all microbes in the natural
world have been grown. Fortunately, there's a better way to
identify organisms not by how they look or how they grow,
but by their DNA.
Identifying protists by their genotype
DNA. We hear a lot about DNA, the genetic
material of all organisms is always in the news. You've probably
heard about DNA fingerprinting to identify suspects in crimes, or
prove paternity. Well, this method of DNA fingerprinting has been
used extensively in the past 15 years or so to identify uncultivated
bacteria and archaea. And, using these new molecular techniques,
we've discovered that the microbial diversity of bacteria and archaea
has been grossly underestimated. Our study, and the rest of the work
I'll present today is the first to look for uncultivated microbial
eukaryotes, or protists, by their DNA fingerprints. How do we do
this?
A tablespoon of mud
We start with about a tablespoon of mud remember that this can contain
several billion microbes. This is mud from Berkeley Aquatic Park. Then we extract the DNA from the mud. By the way, the black color of the mud is a good indicator
that there is no oxygen at that layer. Reduced iron complexed with
sulfur produces something called pyrite a dark black
precipitate.
|
Xeroxing DNA
The polymerase chain reaction, or PCR, is
basically a way to xerox DNA so that we have enough to work with in
the lab. Many microbes are rare and we need to amplify their DNA
signals to be able to find them. In our work we're xeroxing a
specific gene the gene for rRNA. Remember this is what Carl Woese
used to relate different microbes to each other and construct a tree
of life.
Patterns in DNA
Once we amplify rRNA genes from a given
environmental sample, we need to sort them by type. One tablespoon of
mud could have several billion microbes, perhaps representing 1,000 different
types! To categorize these different types, we use a technique called
RFLP (restriction fragment length polymorphism) which generates
unique patterns or fingerprints corresponding to unique organisms.
At right is an example showing the DNA fingerprints of the different types of
sequences (organisms) we find. Notice the different banding
patterns.
Aligning genes
Once we get the DNA sequence, we line up the
sequences and compare them to known organisms. The rows correspond to an
rRNA sequence from a particular organism; the column relates the exact
nucleotide (A,T,C,G) at a specific position in the sequence of the
gene. Note the conserved/variable regions.
Constructing a phylogenetic tree
Then,
from this alignment of DNA rRNA sequences, we use computer models to
simulate the evolution of sequences. We infer that the evolution of
the sequences corresponds to the evolution of the organisms. In this
way, we are able to determine evolutionary relationships among the
new uncultivated microbes. Unlike Linneaus, Darwin or Haeckel, we
organize geneologies based on genotype, not phenotype. This also
gives us a picture of evolution. Note the organisms in blue those
we only know from environmental sequences or fingerprints. Also note
how we can compare where they fall on the tree to known organisms. OK, let's talk more about our study of creatures from the Black Lagoon.
IV. Evolutionary Implications of Uncultivated Eukaryotes
|
Marine Sampling Sites
So we've chosen three sites altogether in which to check for previously
undiscovered microbes. Two of the sites are local: Bolinas
Lagoon by Stinson Beach and the Berkeley Aquatic Park. We chose these two sites because the sediment had low oxygen levels.
Freshwater Sampling
Another site we've investigated is from a
freshwater lake in Bloomington, Indiana. We chose a freshwater site
in addition to the marine sites because they tend to have different
types of protists than marine environments.
So what have we found? Well, lots of types of protists. I've got
plenty of trees, which I'll spare you, but below are some of the
types of organisms we've found. I suppose if we were on their scale,
they truly would be Creatures from the Black Lagoon. These are some
examples of perhaps the most interesting eukaryotic microbes we've
found, especially in the context of the evolution of
eukaryotes.
|
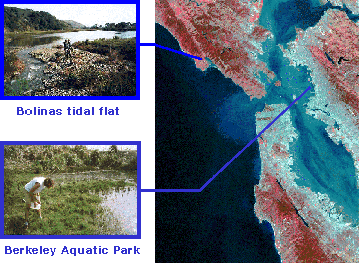
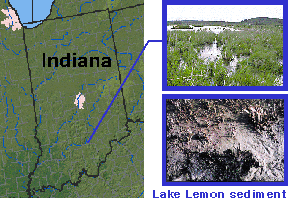
|
Outside the "Crown"
Many of the protists we've found are not closely related to any known
group of protists. The "crown" refers to the most recent "big bang"
in eukaryote evolution where it seems a lot of groups of organisms
evolved quickly, including our animal ancestors. Several of the new
environmental sequences are deep in the tree, suggesting that they
might have retained some characteristics of the ancestral eukaryotes.
We think we've found about eight new kingdoms of eukaryotes in just
three environments by just hunting for their DNA. Not bad for a
teaspoon of stinky mud. These pictures are of Giardia (top left),
Trichomonas (top middle), a slime mold (bottom left), Euglena (bottom
middle) and several amitochondriate amoebae (top and bottom
right).
New Kingdoms of Eukaryotes
Here's a comparison of trees with and
without the new groups of eukaryotes. You'll note both the placement
of the new branches (the mode or pattern of evolution) and the
length of the branches (the rate or tempo of evolution).
Models and Sampling Bias
I think it's obvious that we need to
reconsider our knowledge of extant eukaryotic microbes, those
unseen and ungrown, living today all around us. Adding the few we've
discovered into the tree, it really changes the picture of evolution.
And in coming years, the picture will likely change a lot more.
What's more, we can use our knowledge of the rRNA sequence as a tag
to help identify them if we try to grow them which would be
worthwhile.
Conclusions
In understanding the history of life on
Earth, it is first important to understand our own misconceptions.
So, I've spoken about our own biases and misconceptions in evolution,
life without oxygen, unicellular eukaryotes as opposed to plants and
animals, and how to know organisms without first having to grow
them, and lastly how to find new eukaryotic kingdoms in your own
backyard...
So, it's off to find new microbes where should we look next?
Boiling Hot Springs!
We're thinking that eukaryotes might have adapted to other extreme
environments other than those without oxygen. Maybe somewhere like
this hot spring in Yellowstone National Park pictured at the left.
Aside from being anoxic, the early Earth was also much warmer than
today. The current idea is that Bacteria and Archaea arose during
these hotter times. Why not eukaryotes?
|
|
|